Total body irradiation (TBI) using volumetric modulated arc therapy (VMAT)
VMAT TBI provides a conformal, homogenous dose to the whole body and effective OAR sparing with the patient in a comfortable supine position
Summary
Patient demographics: | Treatment: |
|
|
Diagnosis: | Treatment planning and delivery system: |
|
|
Introduction
The main clinical requirements of high dose total body irradiation (TBI) are: a uniform dose to the whole body with a variation within ± 10%; reduced dose to critical organs, such as the lungs and kidneys; and delivery of radiation dose with a very low dose rate of around 20-40 cGy/minute1-3.
Previously, the most commonly recommended method for TBI was an anterior-posterior/posterior-anterior (AP-PA) technique with the patient positioned in a TBI immobilization device called the TBI stand, using a large field (40 x 40 cm) with 45° collimation and an extended source to surface distance (SSD) of ≥4 meters for better uniform radiation dose distribution. Another common technique was the use of parallel opposed lateral beams. For these methods, photon energies between 6 MV and 10 MV are used with a low dose rate of 15-30 cGy/min1-3. A beam spoiler is used to increase skin dose and partial transmission blocks are used to limit dose to critical organs, such as the lungs and kidneys. The dose is prescribed to the midplane at the level of the umbilicus, to a single point with a uniform dose of ±10% and ±30%, including skin dose. A tissue compensator is recommended for irregular body contours3.
Conventional extended SSD TBI techniques have many limitations and challenges, including: the impact of the large irregular body contour and tissue inhomogeneity on MU calculation and dose estimation accuracy; non-uniform dose distributions; long patient setup and treatment times due to extended SSD and low dose rate treatment; customization of the treatment box and shields; reproducibility of patient setup, including positioning of lung and kidney shields for upright and lateral setups; difficulties for sick patients to stand for a long time (40-50 minutes), which increase setup inaccuracy4-5.
In recent years, TBI has frequently been planned using modulated arc techniques, such as VMAT and TomoTherapy5-9. VMAT-based TBI can be implemented safely and is easily accessible to centers where VMAT is already a standard treatment technique6-9.
VMAT offers a number of advantages for TBI. The patient lies on the couch in a comfortable supine position, which is easier for simulation, improves overall treatment setup, and increases patient comfort. Furthermore, VMAT planning for TBI provides a more conformal and homogenous dose distribution to the target and reduces dose to critical organs, such as the lungs and kidneys (figure 1), and in young patients with benign hematological conditions, reproductive organs. There is no need to customize treatment accessories, such as beam spoilers, treatment frames, and shielding, and it is easy to spare previously treated sites10.
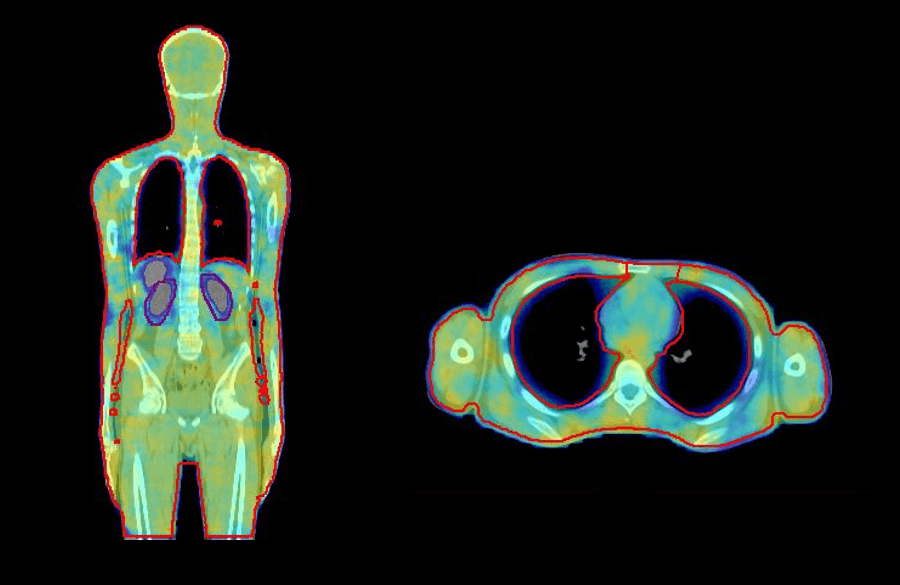
In 2019, we implemented VMAT TBI at our center to overcome the challenges of conventional extended SSD TBI. The following case study demonstrates how we perform accurate and reliable VMAT TBI using Elekta solutions.
Patient history and diagnosis
An 18-year-old male with hyperleucocytosis (Hb-8.2, TLC-1,35,000) presented in March 2019, and was diagnosed with Philadelphia positive B cell acute lymphoblastic leukaemia refractory to BFM-95 protocol. Since the patient’s disease came under control after three cycles of salvage chemotherapy, he was eligible for allogenic Bone Marrow Transplant (BMT). The conditioning regimen for this procedure includes high-dose cyclophosphamide chemotherapy and total body irradiation (12 Gy in 6 fractions, twice daily for three days).
Treatment planning
The patient was simulated for TBI 10 days before BMT on a Discovery IQ (GE healthcare) PET-CT scanner, with a neck rest, a thermoplastic mask for head support, and Elekta BlueBAG™ for body support, using a dedicated TBI CT protocol (table 1). The patient was aligned with lasers and three fiducial markers on the patient’s body (one at head level, a second at chest level, and a third at pelvis level) to ensure reproducible setup for head-first and feet-first setup.
Since this patient was 172 cm in height, he was simulated in head-first supine (HFS) and feet-first supine (FFS) positions. The CT data sets were transferred to Monaco version 5.11.03 for contouring and planning using the Monte Carlo dose calculation algorithm. Delineated structures included the body, lungs, kidneys, liver, eyes, lens, and PTV (cropped 4 mm inside the body and with 3 mm overlap margins with lungs, liver, and kidneys).
Table 1. TBI CT simulation protocol
Patients up to 100 cm length | One planning CT scan data set; head-first supine (HFS); 5 mm slice thickness. |
Patients >100 cm length | Two planning CT scan data sets; head-first supine (HFS) from vertex to lower thigh, up to maximum 150 cm, and feet-first supine (FFS) from toe to pelvis; 5 mm slice thickness. |
Typical VMAT TBI plan details are shown in table 2. In this case, a five-isocenter (aligned over the longitudinal axis) and nine VMAT arc (total) plan was generated and optimized to deliver a uniform dose of 12 Gy in six fractions over three days. The planning aims were to deliver 12 Gy to at least 90% of the PTV (V100% ≥ 90%) and 11.4 Gy to at least 95% of the PTV (V95% ≥ 95%). For the PTV, the mean dose was recorded, as well as the hottest dose to 2 cc (D2 cc) and 5 cc (D5 cc) of the volume as an indicator of maximum dose. The dose homogeneity of the target was reported as the ratio of the dose received by 90% of the volume (D90%) to the minimum dose received by the hottest 10% of the volume (D10%).
Table 2. Typical VMAT TBI plan details
Isocenters |
|
VMAT arcs |
|
Photon beam |
|
Field size/overlap |
|
Calculation parameters |
|
Sequencing parameters |
|
MLC parameters |
|
HFS/FFS |
|
OAR constraints aimed to restrict mean lung dose to < 8 Gy for each lung and combined lung volumes and reduce dose by 15% to the liver and by 30% to the kidneys, while keeping maximum dose and dose homogeneity within acceptable ranges.
During planning phase 1 (theoretical fluence optimization) Multi Criteria Optimization (MCO) was used to decrease OAR dose as much as possible, without compromising the target coverage. Then, in phase 2 (sequencing and segment creation), MCO was toggled off to give Segment Shape Optimization (SSO) more degrees of freedom to converge towards full target coverage.
Dosimetric parameters achieved for this treatment plan are shown in table 3. Although the mean lung doses were slightly higher than plan objectives, they were deemed clinically acceptable. The PTV had an overlap of 3 mm with the lungs to compensate for respiratory motion. Therefore, the higher planning dose covered the peripheral lung, and the central lung was spared.
Table 3. Dosimetric parameters for VMAT TBI plan
Volume | Parameter/criteria | Achieved |
PTV | V100% ≥ 90% V95% ≥ 95% | 90.2% 96.57% |
Homogeneity (D90/D10) | 0.90 | |
D2cc D5cc D10cc | 13.70 Gy 13.60 Gy 13.32 Gy | |
Lungs | Mean dose right lung (< 8 Gy) Mean dose left lung (< 8 Gy) | 8.3 Gy 8.6 Gy |
Kidneys | Mean dose right kidney (reduced by 30%) Mean dose left kidney (reduced by 30%) | 8.2 Gy 7.8 Gy |
Liver | Mean dose (reduced by 15%) | 10.4 Gy |
Quality assurance
Quality assurance for VMAT TBI plans is performed using an Octavius 4D phantom (PTW, Germany). The 3D dose fluence of the VMAT plan is measured for individual isocenters. Dosimetric accuracy between planned and delivered dose is evaluated using gamma index analysis with criteria of 3 mm and 3% in all 3 planes. Chest field QA results for this patient, for the lungs in the coronal plane, are shown in figure 2.
Point dose measurements for all isocenters are also performed, using an Octavius phantom with a 0.07 cm3 Semiflex 3D ion chamber (PTW, Germany). Dose calculation and delivery accuracy between the junctions of isocenters are verified with the point dose measurement at three different points at each junction.
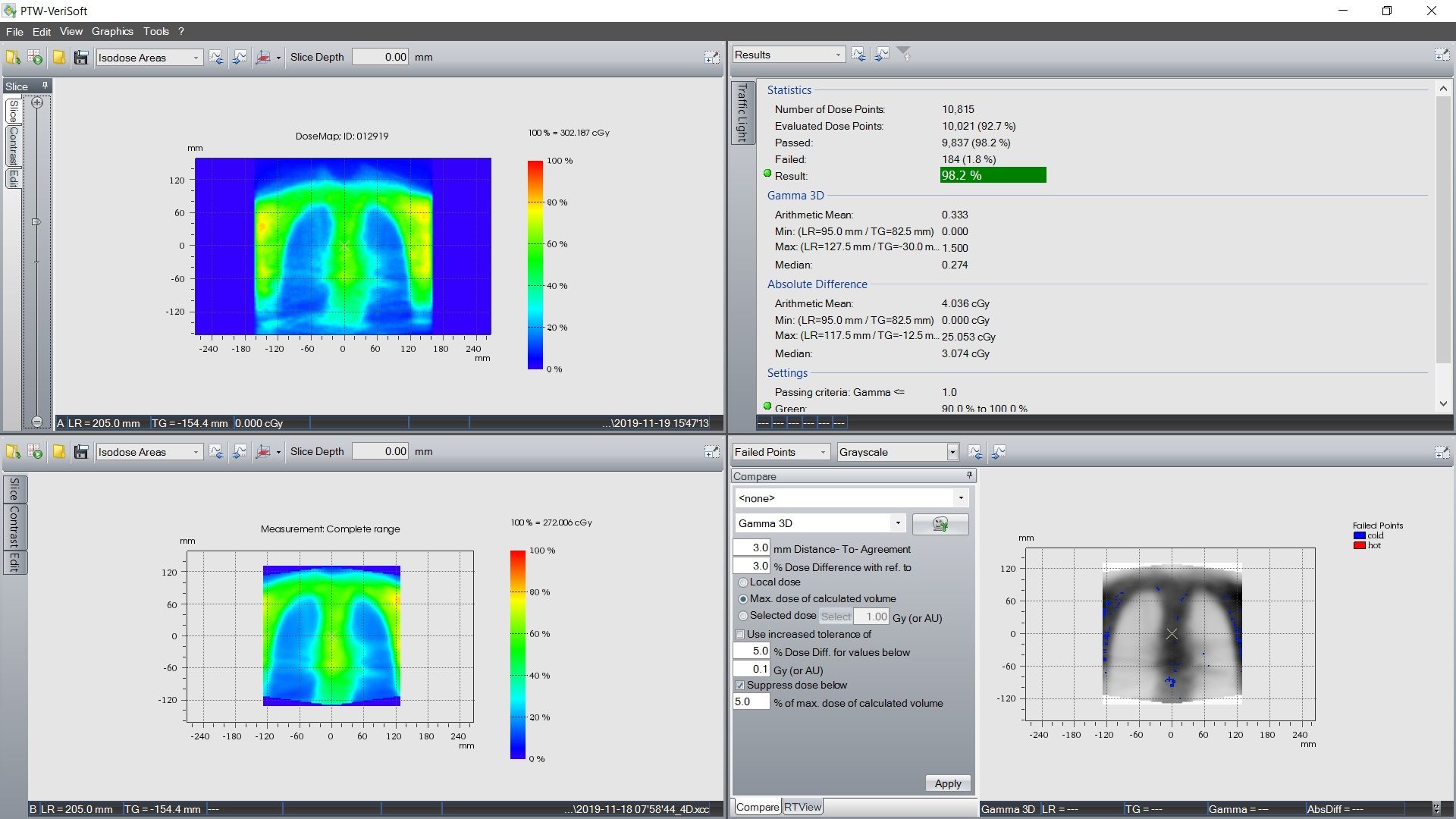
Treatment delivery
The patient was treated twice daily for three days, with a minimum inter-fraction gap of 6 hours. The patient was aligned using the three fiducials and a laser with index setup procedure to ensure reproducibility. Patient setup was verified using XVI 5.0 kV cone-beam CT (CBCT) image guidance and planned kV images for all isocenters before each fraction delivery. Intra-fraction kV imaging was also performed to monitor patient motion during treatment delivery.
The only radiation-associated toxicity noted during and after radiation treatment was nausea (grade 2). Mucositis and dermatitis were not reported.
Outcome and follow up
The patient was successfully treated with TBI and BMT. He recovered and was discharged after one month. The patient is alive and disease-free at D+300, without any significant Graft Versus Host (GVHD), and regularly attends for routine follow-up.
Discussion and conclusions
With the 5 mm leaf width, 40 x 40 cm field size, and effective leaf speed of 6.5 cm/s (combined with the jaw speed of 9 cm/s) of Agility, Monaco effectively optimized the VMAT plan to achieve uniform and homogeneous dose to the large PTV, while restricting OAR dose effectively with the system's virtual 1 mm leaf width capabilities. In addition, since VMAT TBI requires a high number of MUs, Agility’s very low transmission (less than 0.5% for 6 MV) helps to reduce the contribution of transmission dose to the VMAT TBI plan.
Monaco’s XVMC Monte Carlo dose engine calculates the continuous arc as a single beam, rather than approximating dose at many discrete (control point) gantry angle positions. The Monte Carlo algorithm optimizes and calculates dose accurately for tissues of different heterogeneity, such as the lung and PTV interface. By optimizing multiple isocenters in a single optimization, utilizing MCO to reduce OAR dose as much as possible, Monaco reduces planning time and improves overall plan quality. The high-dose junction regions between the two data sets were eliminated by registering two CT data sets using the bias-dose functionality of Monaco 5.11.
VMAT TBI is complex in nature, due to the use of 3-5 isocenters, multiple VMAT arcs, HFS and FFS orientation of the patient for treatment, and its long treatment delivery time. With each of these aspects impacting treatment uncertainty, one of the challenges for this technique is ensuring accurate patient setup. Together with rigid patient immobilization and proper patient marking, setup uncertainties are minimized with pre-treatment and intrafraction kV imaging. The open design and large clearance of Elekta linacs allows pre-treatment and intrafraction imaging to be performed easily without compromising patient setup. The implementation of surface image-guided radiation therapy (SIGRT) can further reduce setup errors caused by intrafraction motion.
TBI using VMAT improves departmental productivity because it is less labour-intensive than conventional TBI. The VMAT method allows the patient to lie in a comfortable supine position, without the need for customized stands or placement of shields, saving significant setup and verification time. XVI imaging is also fast and accurate for treatment setup verification and intra-fraction motion monitoring. Since the average treatment time for VMAT TBI is 40-50 minutes, patient motion monitoring is important for OAR sparing. Intrafraction imaging allows the necessary corrections to be made if shifts are detected during treatment.
The homogenous dose coverage and OAR sparing that can be achieved using VMAT provide confidence to treat patients using complex, whole body, high dose TBI accurately and reliably. Patient outcomes are good, with a maximum of grade 2 toxicity.
It is possible for centers with advanced, VMAT-capable linacs to treat patients using VMAT TBI without modifying the linac bunker. Furthermore, since our radiation oncology staff are very familiar with VMAT treatment planning and delivery, there is no need for special training.
The use of VMAT is currently being evaluated in clinical trials for the delivery of more targeted TBI, referred to as total marrow and lymphoid irradiation (TMLI)11. This technique offers a high degree of dose conformity, dose homogeneity, significantly lower organ doses, reduced toxicity, dose escalation to target structures, and reduced relapse rates. It is hoped that our learning experience with VMAT TBI will help to ease the implementation of VMAT TMLI in our department.
References
- Van Dyk J, Galvin JM, Glasgow GW, Podgorsak E. editors, AAPM report No. 17. The physical aspects of total and half body photon irradiation. American Inst Phys: New York; 1986.
- Carruthers SA, Wallington MM. Total body irradiation and pneumonitis risk: a review of outcomes. Br J Cancer. 2004;90:2080–2084.
- Quast U. Whole body radiotherapy: A TBI-guideline. J Med Phys. 2006;31(1):5-12. doi:10.4103/0971-6203.25664.
- Kirby N, Held M, Morin O, Fogh S, Pouliot J. Inverse-planned modulated-arc total-body irradiation. Med Phys. 2012;39:2761–2764.
- Gruen, Arne, et al. "Total Body Irradiation (TBI) using Helical Tomotherapy in children and young adults undergoing stem cell transplantation." Radiation Oncology 8.1 (2013): 92.
- Chakraborty, Santam, et al. "Total body irradiation using VMAT (RapidArc): A planning study of a novel treatment delivery method." Int J Cancer Ther Oncol 3 (2015): 03028.
- Studinski RCN, Fraser DJ, Samant RS, MacPherson MS. Currentpractice in total-body irradiation: results of a Canada-wide survey.Current Oncol. 2017;24:181.
- Symons K, Morrison C, Parry J, Woodings S, Zissiadis Y. Volumetric modulated arc therapy for total body irradiation: A feasibility study using Pinnacle3 treatment planning system and Elekta Agility™ linac. J Appl Clin Med Phys. 2018;19(2):103-110. doi:10.1002/acm2.12257
- Tas B et al. Total-body irradiation using linac-based volumetric modulated arc therapy: Its clinical accuracy, feasibility and reliability. Radiother Oncol (2018), https://doi.org/10.1016/j.radonc.2018.08.005
- Wong JYC, Filippi AR, Dabaja BS, Yahalom J, Specht L. Total Body Irradiation: Guidelines from the International Lymphoma Radiation Oncology Group (ILROG). Int J Radiat Oncol Biol Phys. 2018;101(3):521-529. doi:10.1016/j.ijrobp.2018.04.071
- Stein, Anthony, et al. "Phase I trial of total marrow and lymphoid irradiation transplantation conditioning in patients with relapsed/refractory acute leukemia." Biology of Blood and Marrow Transplantation 23.4 (2017): 618-624.